A train of Starlink satellites as seen over Japan
Screenshot by Eric Mack/CNET
Ready for Starlink? Are They Ready for You?
A considerable portion of the Philippine population live in remote rural locations classified as geographically isolated and disadvantaged areas (GIDAs) that continue to remain unconnected to the Internet. The lack of mobile cellular networks and fixed wired or wireless Internet services in these areas remains a persistent and serious problem. For people residing in GIDAs, the best alternative they might have for now to be connected online is through satellite-based Internet. In such scenarios, large geostationary (GEO) Internet satellites have traditionally served as a means to deliver Internet services quickly, with only minimal ground infrastructure required to be set up. In recent years, a few groups have been gaining traction in championing the use of small satellite mega-constellations in low Earth orbit (LEO) to deliver broadband Internet, which we shall discuss in this piece.
Emerging LEO Satellite Internet Solutions
A mega-constellation consists of hundreds to thousands of satellites deployed and spread out in orbit to provide extensive and continuous coverage. LEO mega-constellations are designed to orbit at altitudes much closer to Earth, i.e. less than 1,200 km above the ground compared to ~36,000 km for GEO counterparts. The most well-known systems, either proposed, currently under development or pilot testing, include Starlink (of SpaceX), OneWeb, Telesat LEO, CurvaNet and Kuiper (of Amazon). The closer proximity means that the delay associated with the travel time of the radio wave signal from the Earth to the satellite and back, i.e. roundtrip travel time or RTT, is much smaller at ~50 milliseconds (ms), compared to ~500 ms for those in GEO orbit. The smaller “lag time” expected in LEO systems can better support applications demanding near real-time response or lower latency such as interactive gaming and video teleconferencing. This capability for reduced latency offers an advantage and value proposition that can set LEO Internet constellations apart in the telecommunications satellite industry. The system design, technologies, operational and business models of these companies have also been geared towards addressing the long-standing needs of unserved and underserved populations and, as such, have rightfully gained significant global attention (perhaps now at a peak of inflated expectations). If proven effective in the long run, these systems are poised to transform not only the satellite broadband industry but the telecommunications industry in general.
As a consequence of their orbit characteristics, individual LEO satellites keep moving in space relative to the Earth so their “footprint” or coverage on the ground is also not fixed. This is unlike satellites in geostationary (GEO) orbit whose higher altitude and consequently synchronous revolution with the Earth’s rotation makes them appear stationary in the sky. This explains why it is necessary to deploy a larger number of LEO satellites to span the whole planet at all times. Thus, there is a stark contrast between GEO and LEO satellite broadband systems: to achieve global coverage, GEO systems need only a few large spacecraft designed to last for around 15 years, while LEO systems require hundreds to thousands of small spacecraft each needing replacement every five years or so. In the LEO approach, many compact satellites are distributed in space to deliver coverage and capacity comparable to what a few massive GEO satellites can accomplish. Therefore, to set up the space infrastructure, GEO systems would involve only a few but larger rocket launches, while LEO systems would need more regular, albeit can be relatively smaller, rocket launches to be conducted.
The rise of LEO systems has also helped spur the development and adoption of innovative ways of doing things in the space industry, including launch technologies such as micro-, small, medium launchers, and large launchers packed with many satellites; as well as spacecraft and constellation design and deployment. In particular, some companies have developed unprecedented systems for rapid and cost-effective assembly, integration and testing (AIT) of multiple small satellites.
LEO satellite broadband services are not yet available in the Philippines but it is important to create more awareness about these emerging technologies and how they can address the continuing digital divide in the country. Currently deployed LEO constellations for satellite broadband do pass over the Philippines. However, they do not operate because they do not have a license to transmit at this time. In the following, we focus on the Starlink LEO system and share our results on simulating its “coverage” or “availability” over the Philippines.
Brief Information on the Starlink System
Starlink, the satellite Internet company developed by SpaceX, has over 1,000 satellites orbiting the Earth as of this writing. Their goal is to provide Internet access to all parts of the world that would greatly benefit the unserved and underserved areas. These small satellites, each weighing approximately 260 kg, are part of the planned constellation of 4,409 satellites flying at 540-570 km altitude and operating in Ku/Ka-band [a]Ku-band and Ka-band are designated portions of the electromagnetic spectrum with a range of frequencies within 12-18 GHz and 26.5-40 GHz, respectively. frequencies, which the US Federal Communications Commission (FCC) approved [1]. In addition, they have also secured approval for a 7,518-satellite constellation operating at 335-346 km (i.e., Very LEO or VLEO) using V-band frequencies [b]V-band is within the range of 40-75 GHz.. Starlink has been gearing to launch about 120 satellites every month on its way to complete the constellation of approximately 12,000 small satellites [2].
Starlink has been rolling out their service starting with the US and Canada and is planning to expand services to other countries “as soon as [they] receive regulatory approval” [3]. In August 2020, Starlink was granted a license by the Australian Communications and Media Authority (ACMA) to offer satellite Internet services in Australia, which may be offered sometime in 2021 [4]. In a bid to accommodate further demand, they filed a new petition at FCC in June 2020 seeking approval for a second-generation system consisting of 30,000 satellites with altitudes ranging from 328 km to 614 km and using Ku/Ka-band frequencies [5].
Meanwhile, initial reports from beta testers have noted “impressive” overall performance despite the occasional unstable link, jitter, and variable speeds [6]. In order to tone down the expectations of its beta test participants, Starlink cautioned that hiccups, including “brief periods of no connectivity at all”, are expected during the “Better Than Nothing” beta test period as it is still building up its satellite constellation, ground segment and also fine-tuning the network settings. Another article [7] cited a presentation by SpaceX to FCC in September 2020, highlighting that using their standard user terminal equipment, they had achieved over 100 Megabits per second (Mbps) downlink and 42 Mbps uplink speeds with latencies below 40-50 ms round trip to the Internet. For perspective, a delay or latency of more than 200 ms during voice or video calls is perceptible by humans and can degrade the quality of the communication and therefore the user experience. We also note that this downlink speed is much higher than the average Internet download speed of 18.49 Mbps (mobile) and 28.69 Mbps (fixed) in the Philippines reported by the National Telecommunications Commission (NTC) [8].
Starlink’s user terminal equipment is capable of continuously tracking the passing satellites. Their design leverages phased array antenna technology to implement electronic multiple-beam steering that does not require mechanical movement for tracking the satellites. All this requires fairly ingenious engineering design and development work, which Starlink has managed to do at a cost of $499 per terminal [c]Includes the phase array antenna, satellite modem, mount and Wi-Fi router to the consumer. This cost is on top of the $99 per month subscription fee, at least during the period of its public beta tests. We note that these prices apply to current trials in the US and is likely subject to change in other geographic service areas.
As the Starlink system operates in LEO, they need to deploy a vast number of satellites to provide wide geographical coverage. One of the merits mentioned by SpaceX to justify relocating succeeding satellites at lower altitudes is that, at roughly 550 km orbit, satellites will de-orbit within five years (pages 6-7 in [1]), which also attempt to address concerns on orbital congestion and space debris (for additional reading on space debris, please see references [9] and [10]). In this low Earth orbit, a satellite would only be accessible from an observer on the ground for a few minutes. Thus, to provide consistent Internet connectivity, there should be one or more passing satellites that provide coverage to the users at any given time. In other words, these satellites should have overlapping coverage over a user location to facilitate seamless handover procedures. Like the Starlink satellites, the Philippine microsatellites, Diwata-1 and Diwata-2 and the nanosatellite Maya-1 were also deployed in LEO, passing by our country around 2-4 times a day with a communication window of about 10 minutes at a fixed location. Figure 1 shows a simplified illustration of the tracking and handover between satellites to ensure continuous connection (we note that this is for illustration purposes only and may not accurately reflect actual operation of the Starlink system). To be connected, there should be at least one satellite within the Field of View (FOV) and an uninterrupted connection requires seamless and timely handoff to the next satellite passing over the horizon. A phased array antenna uses electronically steered (multiple) beams to track satellites and perform the handoff.
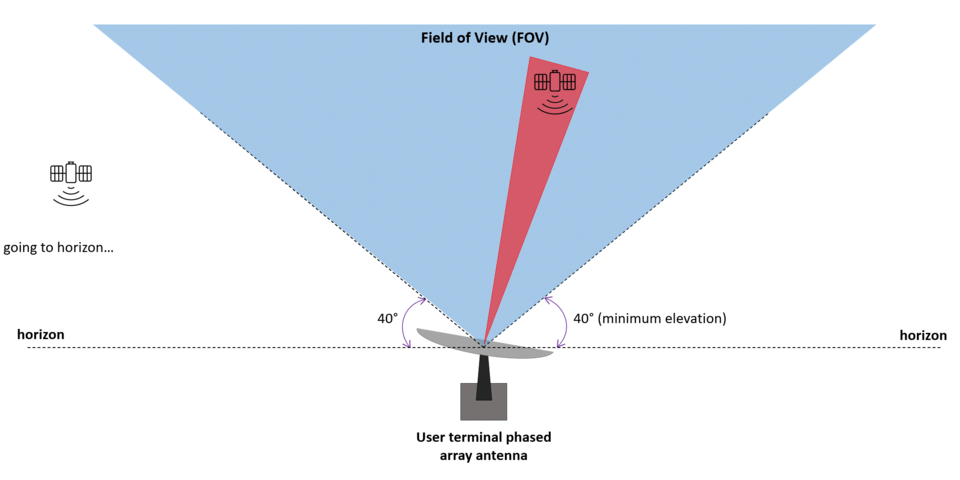
Figure 1. Simplified illustration of a user terminal’s phased array antenna tracking contiguous satellite passes.
Starlink Satellite Coverage Simulation
Figure 2 shows a visualization of the Starlink satellites as they orbit around the Earth using a free orbital and satellite-tracking software called Orbitron. Interested readers may refer to the tutorial at the end of this article to learn how to simulate satellite passes using the software. A satellite “train” is also depicted as it passes over the Philippines. According to one article [11], this train of satellites can be seen in different parts of the world. It became a spectacle that led people to use apps and different tools to check when they can spot it and observe its fly-by. While it became a night sky show for some people, astronomers are complaining that this has caused light pollution. This prompted Starlink to make some design changes to address this concern. It was reported in another article [12] that they have implemented a software upgrade so that the satellites will fly with their “knife-edge” to the sun to reflect less light towards the Earth. Succeeding satellites will also be launched with a visor to prevent sunlight from hitting the brightest parts of the spacecraft.

Figure 2. Orbital simulation of Starlink satellites using Orbitron software
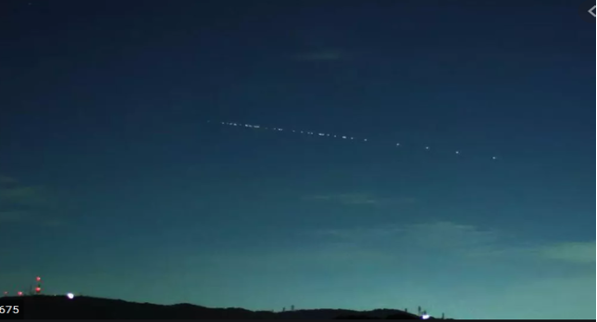
Figure 3. A train of Starlink satellites as seen over Japan
Screenshot by Eric Mack/CNET
Although Starlink Internet service is not yet available in the Philippines, these satellites do pass over our country as shown in Figure 2. Using simulations, we determine the number of satellites that are “visible” or “available” from a particular ground location in the Philippines, considering the constellation of 890 satellites as of December 2020 and, for comparison, the 1017 satellites as of this writing in February 2021. By doing so, we could arrive at an estimate or a picture of the “coverage” or “availability” at various points in the country if the constellation were to start operating in our territory at this time.
Using the latest two-line elements (TLE) of Starlink satellites from Celestrak [d]CelesTrak maintains an updated online catalog of satellites and Two Line Elements sets according to the surveillance data on space objects from the North American Aerospace Defense Command (NORAD). … Continue reading , we developed a Python script to run a simulation modeling the orbits of the satellites and to compute the coverage over selected ground user terminal locations. Using Orbitron software, we can set the location of a ground user terminal and make use of the latest two-line elements (TLE) from CelesTrak to run a simulation modelling the orbits of the satellites. We consider a satellite as “available” when it is above a minimum elevation of 40° from the horizon, i.e. the “Field of View” (FOV). This requirement is based on the basic technical description of the Starlink antennas as indicated in their FCC filings [13]. For simplicity, the simulation does not account for other components related to networking, radio propagation, access limitations or prioritization, inoperative satellites, and other details
A. Quezon City (QC), Philippines (14.46475°N, 121.072°E)
Figures 4, 5, and 6 show the simulation results for a location in Diliman, QC over a 24-hour period from December 11-12, 2020 (890 satellites) and from February 15-16, 2021 (1,017 satellites).
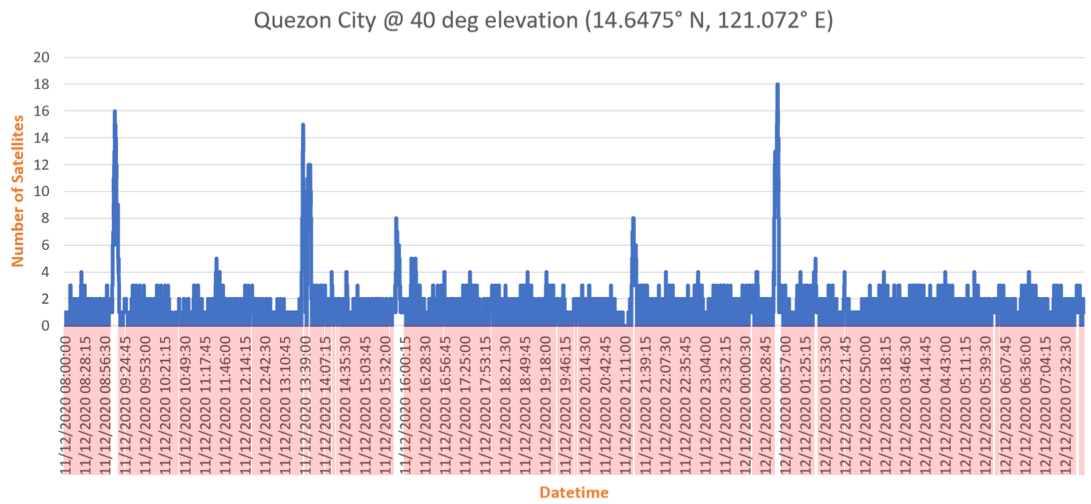
(a) 24-hour period from December 11-12, 2020 with 890 Starlink satellites in the constellation, Quezon City. Red lines indicate the 275 instances with zero satellites (at 40° minimum elevation).
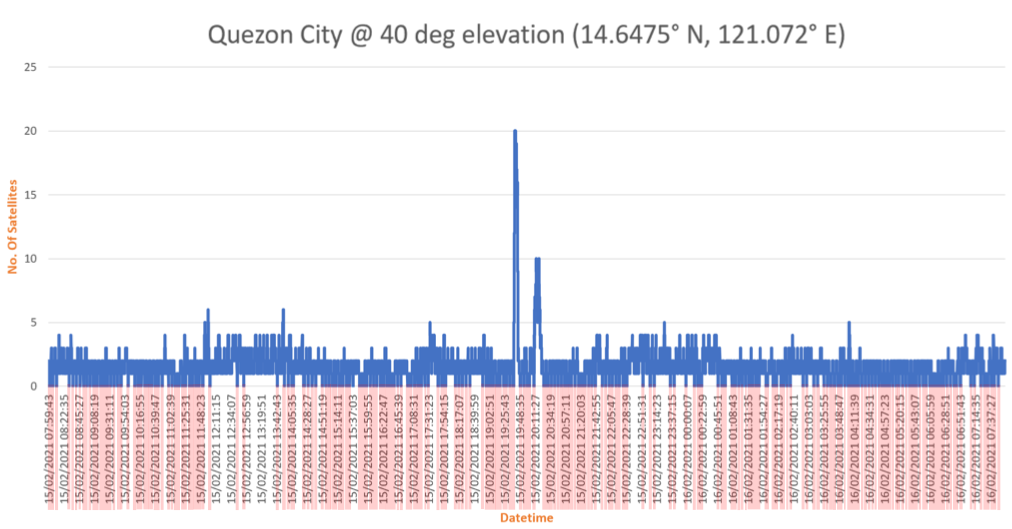
(b) 24-hour period from February 15-16, 2021 with 1,017 Starlink satellites in the constellation, Quezon City. Red lines indicate the 221 instances with zero satellites (at 40° minimum elevation).
Figure 4. Number of “available” Starlink satellites simulated over a 24-hour period and 40° minimum elevation, Quezon City (14.6475° N, 121.072° E).
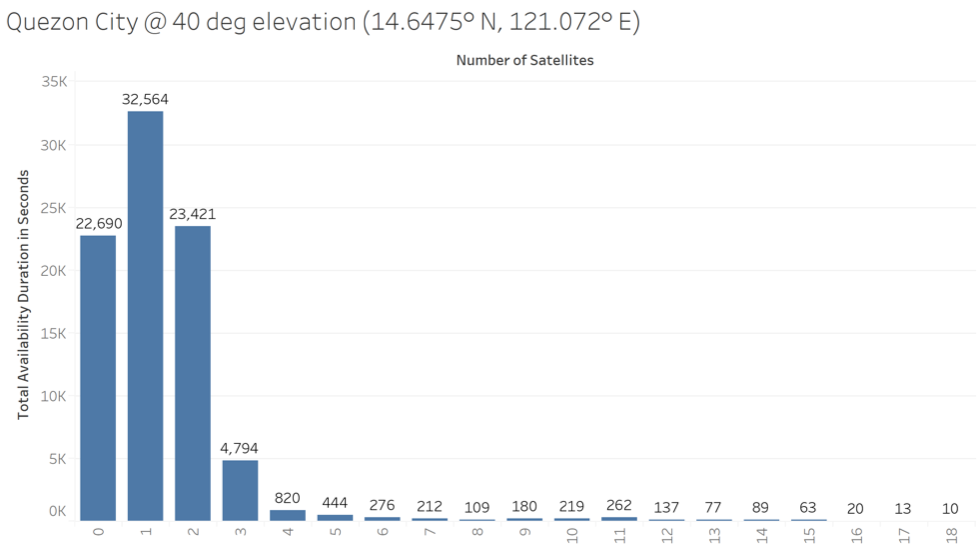
(a) December 11-12, 2020 with 890 Starlink satellites in the constellation, Quezon City. There are 22,690 seconds (~6.3 hours) with zero satellites (at 40° minimum elevation).
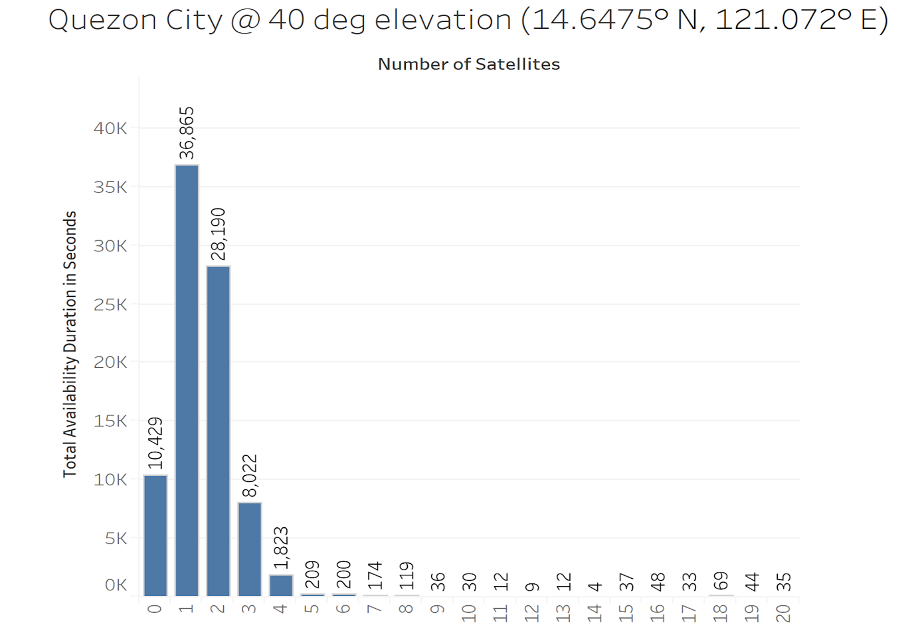
(b) February 15-16, 2021 with 1,017 Starlink satellites in the constellation, Quezon City. There are 10,429 seconds (~2.9 hrs) with zero satellites (at 40° minimum elevation).
Figure 5. Histogram showing the duration of “availability” in seconds vs. different number of Starlink satellites in Quezon City (14.6475° N, 121.072° E) over a 24-hour period and 40° minimum elevation.
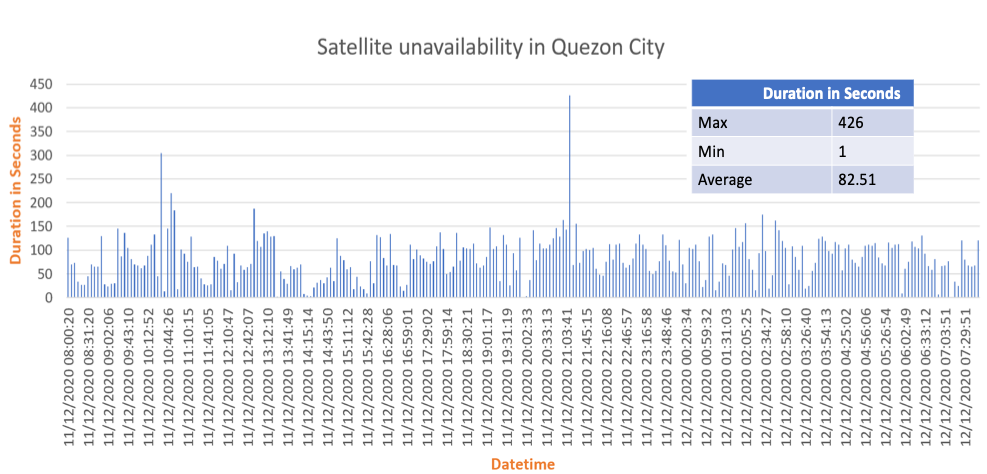
(a) Distribution and duration of the 275 instances with zero satellites (at 40° minimum elevation) in Quezon City over the 24-hr period, 11-12 December 2020 (with 890 Starlink satellites in the constellation). The peak continuous duration is 426 seconds (7.1 mins).
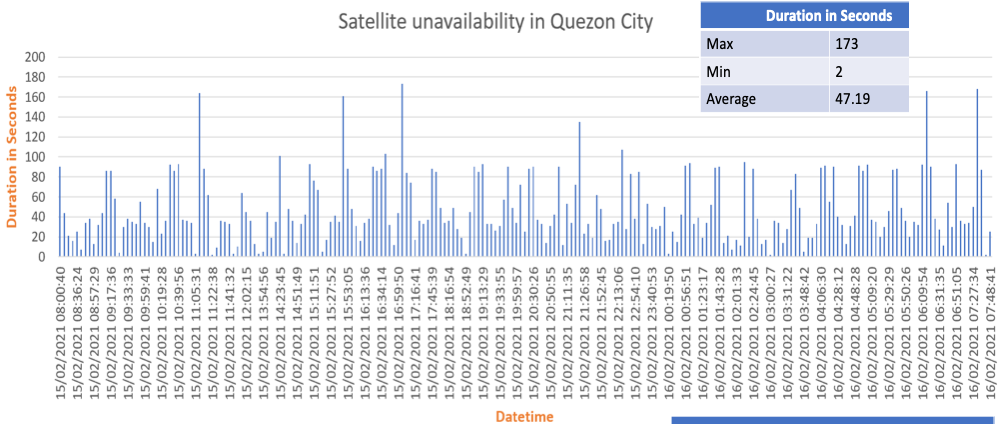
(b) Distribution and duration of the 221 instances with zero satellites (at 40° minimum elevation) in Quezon City over the 24-hr period, 15-16 February 2021 (with 1,017 Starlink satellites in the constellation). The peak continuous duration is reduced to 173 seconds (2.88 mins).
Figure 6. Instances of zero satellites and their durations simulated over a 24 hour period and 40° minimum elevation, Quezon City (14.6475° N, 121.072° E).
B. Availability over Marawi City (MC), Philippines
We also simulated the Starlink passes over Marawi City in Mindanao. Figures 7, 8 and 9 shown below are the simulation results over the same dates.
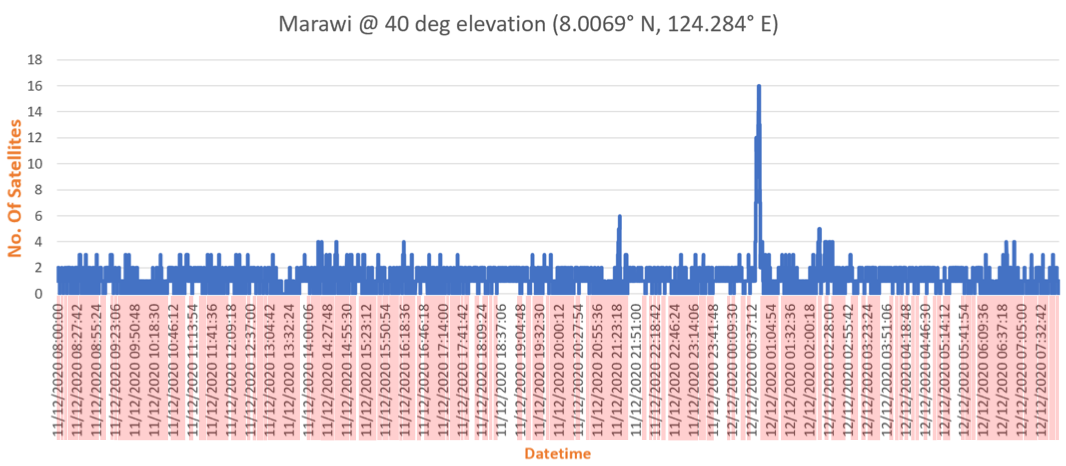
(a) 24-hour period from December 11-12, 2020 with 890 Starlink satellites in the constellation, Marawi City. Red lines indicate the 229 instances with zero satellites (at 40° minimum elevation).
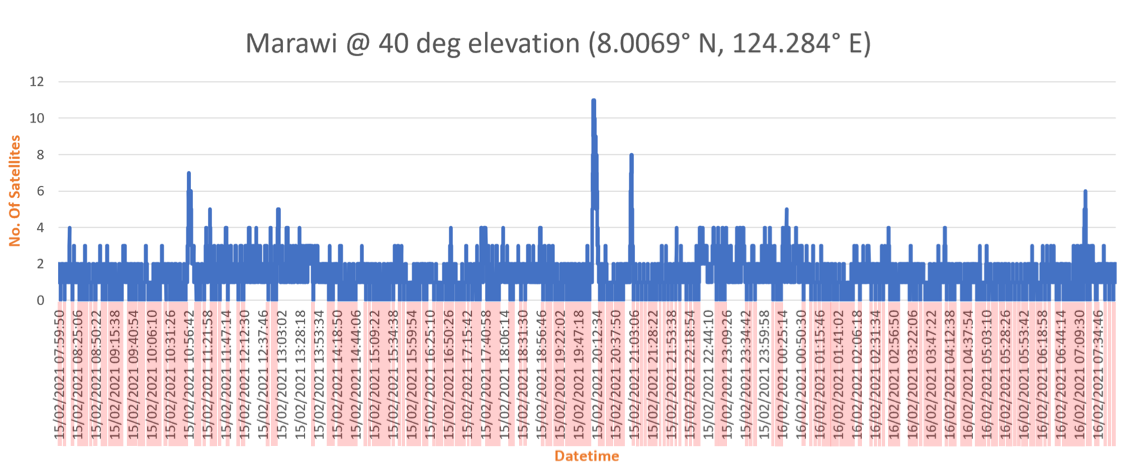
(b) 24-hour period from February 15-16, 2021 with 1,017 satellites in the constellation, Marawi City. Red lines indicate the 209 instances with zero satellites (at 40° minimum elevation).
Figure 7. Number of “available” Starlink satellites over a 24-hour period and 40° minimum elevation, Marawi City (8.0069° N, 124.284° E).
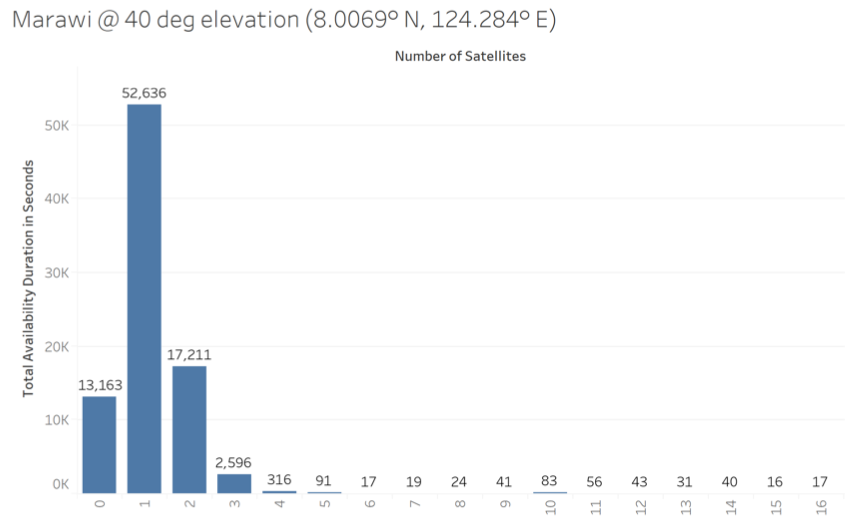
(a) December 11-12, 2020 with 890 Starlink satellites in the constellation, 40° minimum elevation, Marawi City. There are 13,163 seconds (~3.66 hrs) with zero satellites (at 40° minimum elevation).
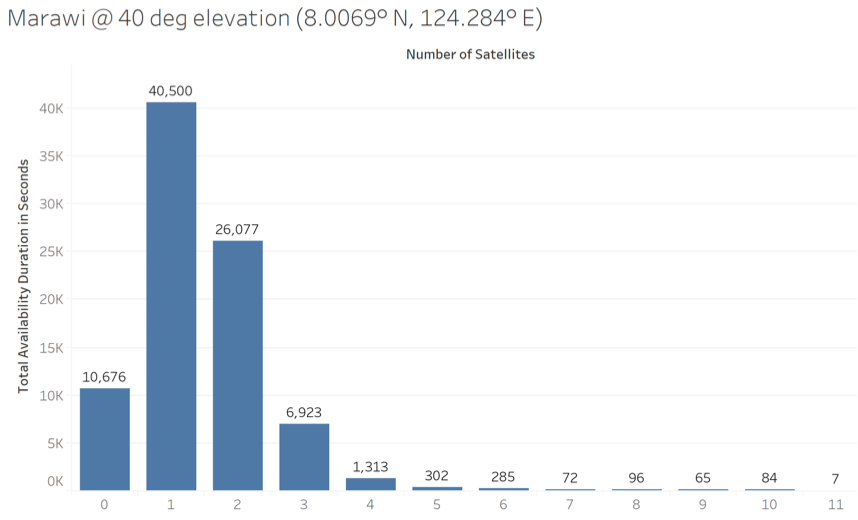
(b) February 15-16, 2021 with 1,017 satellites in the constellation, Marawi City. There are 10,676 seconds (2.96 hrs) with zero satellites (at 40° minimum elevation).
Figure 8. Histogram showing the duration of “availability” in seconds vs. different number of satellites in Marawi City (8.0069° N, 124.284° E) over a 24 -hour period and 40° minimum elevation.
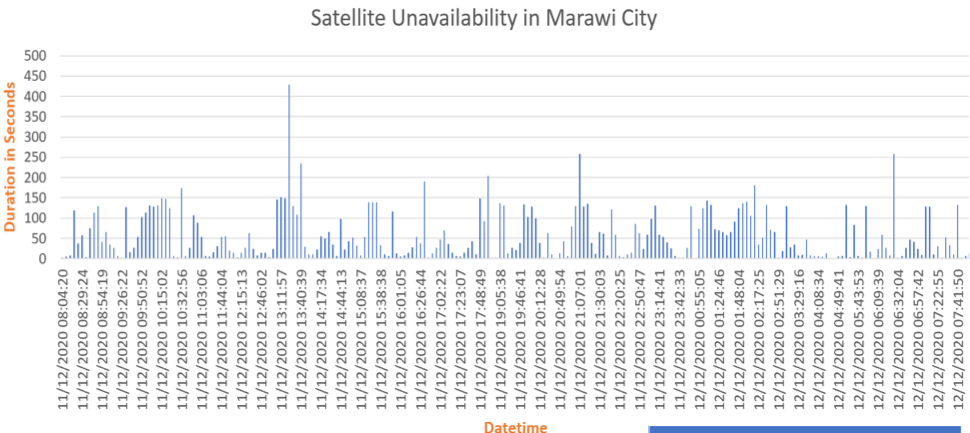
(a) Distribution and duration of the 229 instances with zero satellites (at 40° minimum elevation) in Marawi City over the 24-hr period, 11-12 December 2020 (with 890 Starlink satellites in the constellation). The peak continuous duration is 429 seconds (7.15 mins).
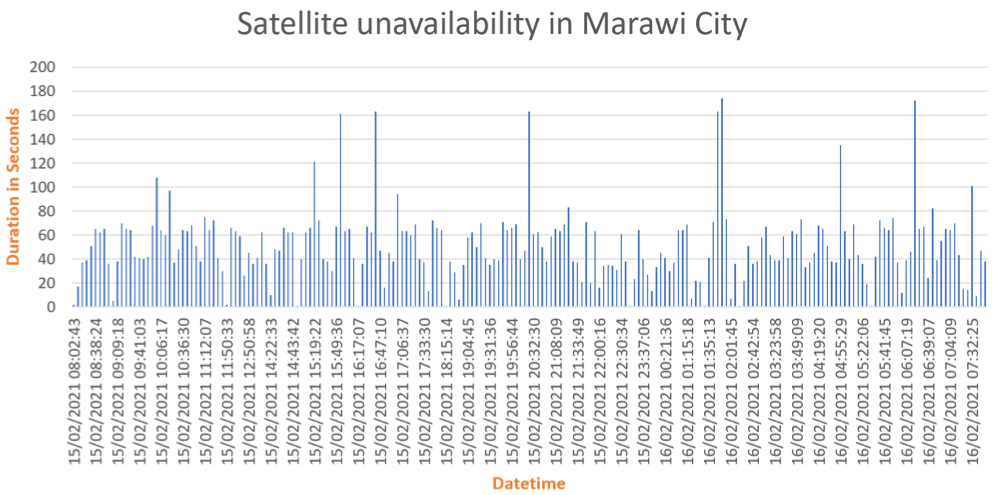
(b) Distribution and duration of the 209 instances with zero satellites (at 40° minimum elevation) in Marawi City over the 24-hr period, 15-16 February 2021 (with 1,017 Starlink satellites in the constellation). The peak continuous duration is reduced to 174 seconds (2.88 mins).
Figure 9. Instances of zero satellites and their durations simulated over a 24 hour period and 40° minimum elevation, Marawi City (8.0069° N, 124.284° E).
C. Availability over Toronto, Canada
For comparison, we performed the same simulation for the case of a fixed terminal located in Toronto, Canada over the same time periods. We should note that the Starlink satellite Internet service is already conducting trials in the said area. It is plausible that the existing constellation has been initially configured to provide better coverage over higher latitude regions such as those in the continental US and Canada, which were the original targets of the early trials.
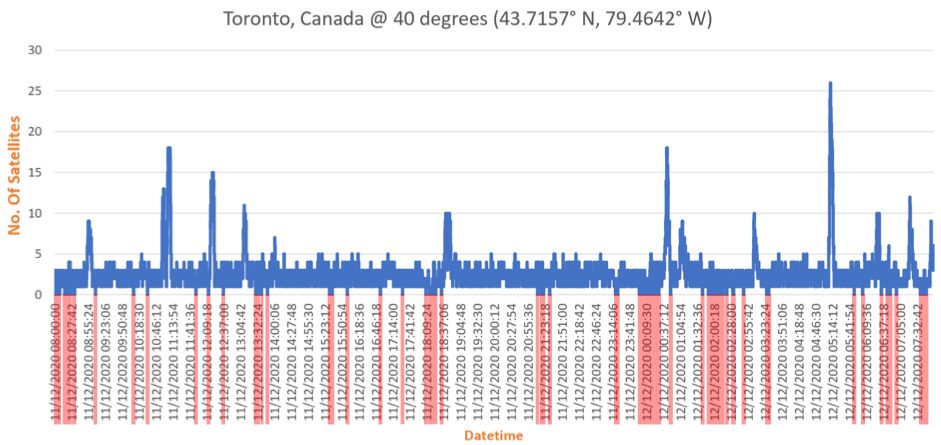
(a) 24-hour period from December 11-12, 2020 with 890 Starlink satellites in the constellation, Toronto, Canada. Red lines indicate the 67 instances with zero satellites (at 40° minimum elevation).
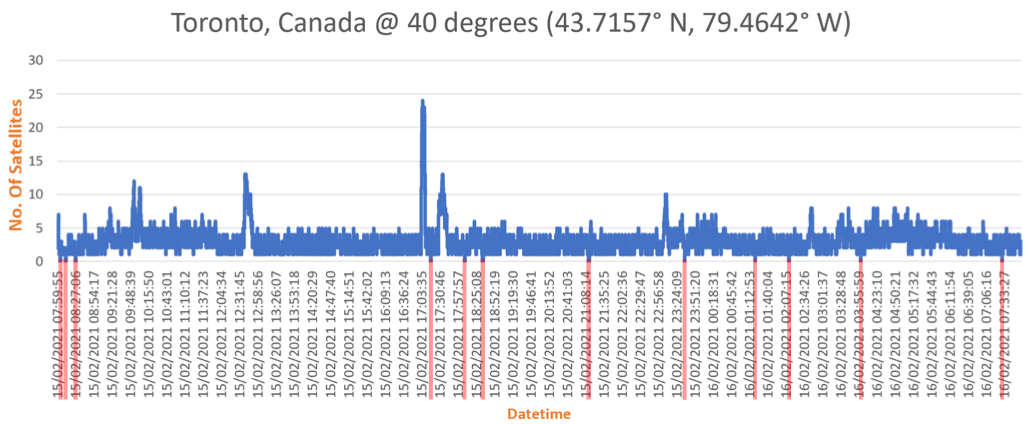
(b) 24-hour period from February 15-16, 2021 with 1,017 Starlink satellites in the constellation, Toronto, Canada. Red lines indicate the 12 instances with zero satellites (at 40° minimum elevation).
Figure 10. Number of “available” Starlink satellites simulated over a 24-hour period and 40° minimum elevation, Toronto, Canada (43.7157° N, 79.4642° E).
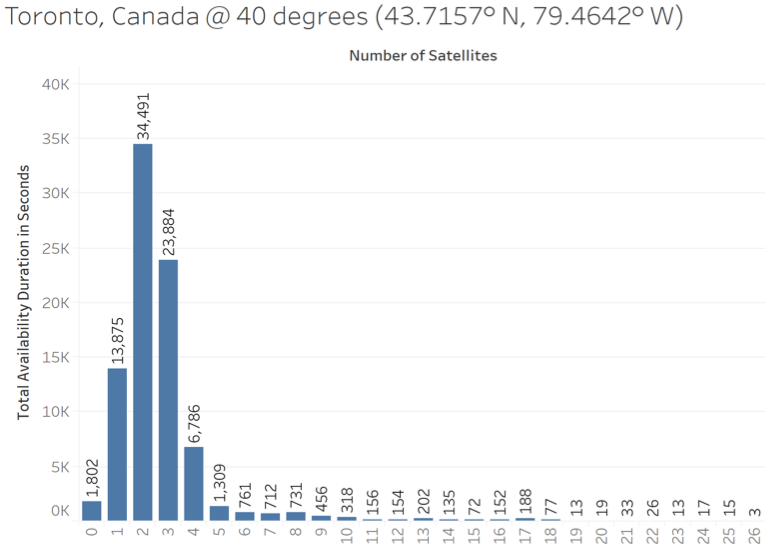
(a) December 11-12, 2020 with 890 Starlink satellites in the constellation, Toronto, Canada. There are 1,802 seconds (~30 mins) with zero satellites (at 40° minimum elevation).
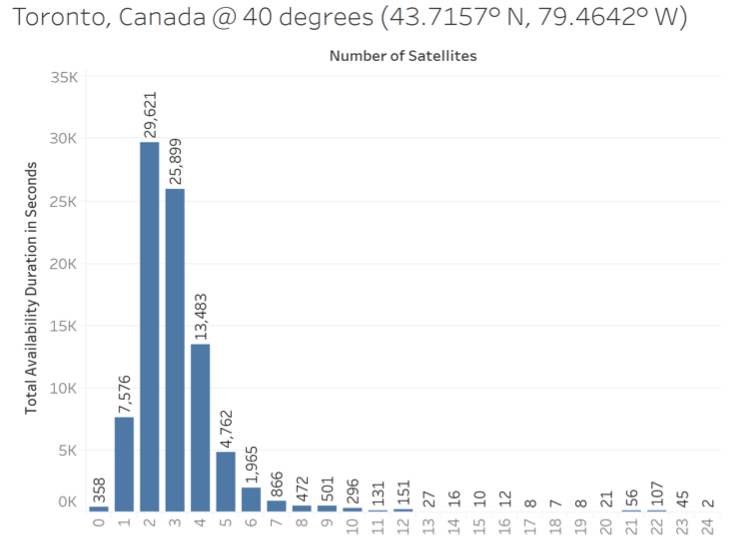
(b) February 15-16, 2021 with 1,017 Starlink satellites in the constellation, Toronto, Canada. There are 358 seconds (~5.97 mins) with zero satellites (at 40° minimum elevation).
Figure 11. Histogram showing the duration of “availability” in seconds vs. different number of satellites in Toronto, Canada (43.7157° N, 79.4642° E) over a 24 -hour period and 40° minimum elevation.
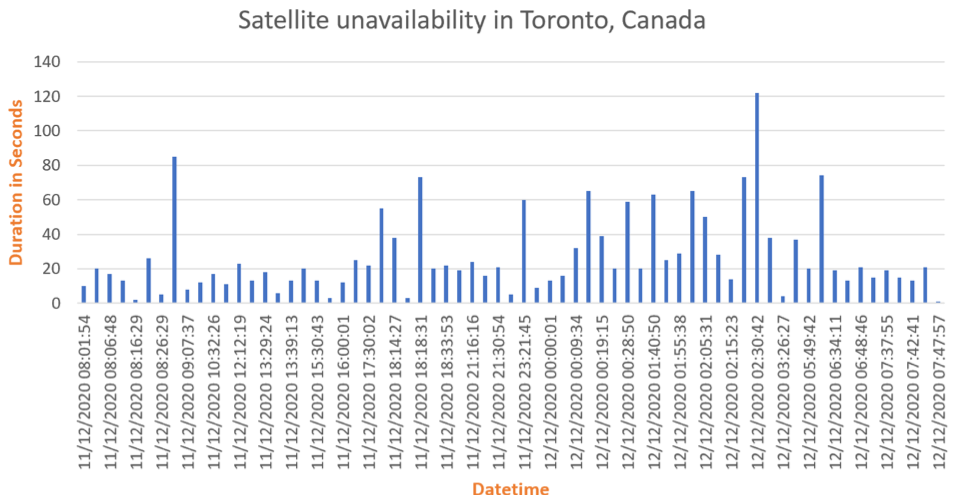
(a) Distribution and duration of the 67 instances with zero satellites (at 40° minimum elevation) in Toronto, Canada over the 24-hr period, 11-12 December 2020 (with 890 Starlink satellites in the constellation). The peak continuous duration is 122 seconds (~2 mins).
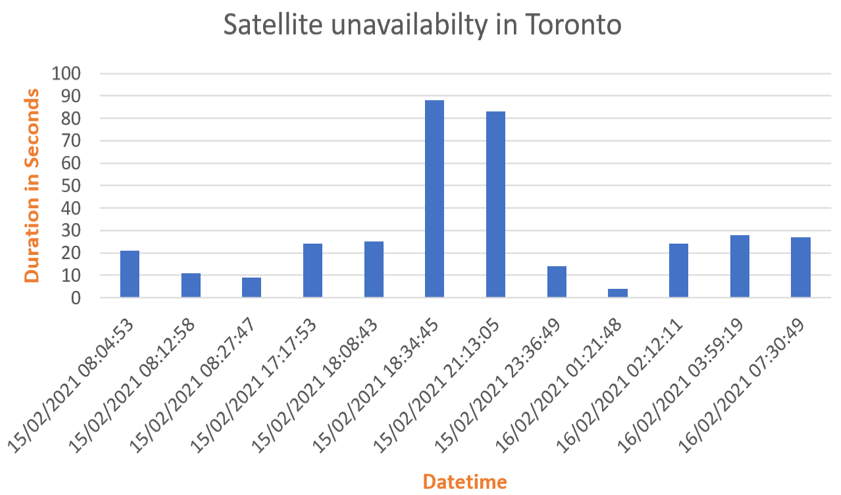
(b) Distribution and duration of the 12 instances with zero satellites (at 40° minimum elevation) in Toronto, Canada over the 24-hr period, 15-16 February 2021 (with 1,017 Starlink satellites in the constellation). The peak continuous duration is reduced to 88 seconds (~1.47 mins).
Figure 12. Instances of zero satellites and their durations simulated over a 24 hour period and 40° minimum elevation, Toronto, Canada (43.7157° N, 79.4642° E).
The highlights of the 24-hour simulation data for Quezon City, Marawi City and Toronto, Canada for the two periods considered: 11-12 December 2020 and 15-16 February 2021 are summarized in Table 1. The Total Period with Zero Satellites (TPZS) is the aggregate of all instances where there are no satellites within the FOV while the Peak Duration with Zero Satellites (PDZS) refers to the maximum continuous duration of any such instance over a 24-hour period.
Table 1. Summary of simulation results of Starlink satellite “availability”
|
11-12 December 40° minimum elevation 890 satellites
|
15-16 February 40° minimum elevation 1,017 satellites |
||||
|
Quezon City (14.6475°N, |
Marawi City (8.0069°N, |
Toronto, Canada (43.7157°N, |
Quezon City (14.6475°N, |
Marawi City (8.0069°N, |
Toronto, Canada (43.7157°N,
|
Total Period with Zero Satellites (TPZS) (over the 24-hr period) |
22,690 (~6.3 hrs) |
13,163 (~3.66 hrs) |
1,802 (~30 mins) |
10,429 (~2.9 hrs) |
10,676 (~2.96 hrs) |
358 (~5.97 mins)
|
Percentage “availability” (over the 24-hr |
~73.7% |
~84.7% |
~97.9% |
~87.9% |
~87.6% |
~99.5% |
Number of distinct instances with zero satellites (over the |
275 |
229 |
67 |
221 |
209 |
12 |
Peak Duration with Zero Satellites (PDZS) (over the 24-hr period) |
426 secs (~7.1mins) |
429 secs (~7.15mins) |
122 secs (~2mins) |
173 secs (~2.9mins) |
174 secs (~2.9mins) |
88 secs (~1.47mins) |
Average Period with Zero Satellites (APZS) (over the 24-hr period) |
82.51 secs |
57.48 secs |
26.9 secs |
47.19 secs |
51.08 secs |
29.83 secs |
We make the following observations from the summary in Table 1:
- Between 11-12 December 2020 and 15-16 February 2021, the number of instances with no satellites above 40° minimum elevation has decreased across the three (3) sample locations considered. This is expected with the insertion of more satellites into orbit, from 890 to 1,017 in this case, which tends to increase the frequency of visits (and/or “revisits”) over an area.
- The TPZS was reduced by around 54% and 18.8% for Quezon City (QC) and Marawi City (MC), respectively. The TPZS reduction is more pronounced in Toronto, Canada (TC) at around 80%, likely owing to the prioritization of this region due to the ongoing service trials.
- Despite the higher reduction level of TPZS in QC, the absolute level (~2.9 hrs) is roughly the same as that in Marawi (~2.96 hrs) for 15-16 February 2021. For perspective, the TPZS in Toronto, where trials are ongoing, are at a mere ~5.97 minutes, indicating the disparity in the “availability” level between the locations in Canada and in the Philippines.
- The 12 instances of zero satellites in TC for 15-16 February 2021 is quite sparse and contrasts sharply with the 221 and 209 instances for QC and MC, respectively, which are distributed across numerous times in the day. These instances translate to gaps in connectivity that can degrade the user experience. Service providers recognize the incessant demand for connectivity and therefore aim to minimize the occurrence of interruptions, downtimes or outages.
- The PDZS between the two time periods has also decreased for all sample locations, i.e. approximately 59% for both QC and MC and 27.8% for TC. This is also a desired and expected effect of the increase of the satellite constellation size.
- While the TPZS levels differ significantly between Toronto and QC/MC, the PDZS levels are much closer. For the TPZS, the level in QC or MC are roughly 30x that in Toronto for 15-16 February 2021. For the PDZS, however, the level in QC or MC is only around 2x that in Toronto for the same period.
- Despite the decrease in both the TPZS and PDZS in QC and MC, the absolute level of availability is still low when compared to TC. Based on the TPZS, the percentage of “availability” for 15-16 February 2021 is at 87.9% and 87.6% for QC and MC, respectively. On the other hand, the Starlink trials that were ongoing over Toronto, Canada back in December 2020 already had 97.9% “availability” level. This can perhaps give an indication of the level that needs to be reached in any prospective location before trials can begin to happen there.
Summary
Our simulation results of the Starlink LEO Internet satellite constellation are intended to offer a window into their “availability” in the country. The parameters “Total Period with Zero Satellites” (TPZS) and “Peak Duration with Zero Satellites” (PDZS) are defined respectively as the aggregate and the single longest duration of the instances with no satellites within a chosen FOV over a 24-hr period. We chose a FOV based on a minimum elevation of 40° for our simulations, which may be considered conservative. We note that we had also conducted simulations at 25° minimum elevation that yielded different (i.e. more favorable) TPZS and PDZS results over the Philippines. Within the chosen FOV, we also assume that there is clear line-of-sight (LOS) between the ground terminal and the passing satellite[e]High-rise buildings, trees or other objects may come within the FOV and obstruct the signal path as the ground terminal antenna tracks the passing satellite. Choosing a wider FOV, e.g. 25° minimum … Continue reading. Our simulation used a time resolution of 1 second, which means that we are unable to track any changes in the movement of the satellites and, consequently, their presence within the FOV at time scales less than that. Considering that a LEO satellite at ~500km altitude passing overhead at 40° elevation will complete a pass in roughly 2.5 minutes, we consider a time granularity of 1 second as practical. We chose fixed points in Quezon City (QC), Marawi City (MC) and Toronto, Canada (TC) as representative locations for ground terminals. Given that the initial trials of the Starlink Internet connectivity were aimed at the northern latitude regions in the continental US and Canada, the juxtaposition of the results from QC and MC with TC may provide some insight into Starlink’s level of “service readiness” in our country. In particular, the “availability” levels in Toronto may give an indication of what to expect when trials (or full service) eventually commence in the Philippines.
The results over QC and MC reveal an “availability” of 87.9% and 87.6%, respectively, over 15-16 February 2021 with 1,017 satellites in the Starlink constellation, compared to 73.7% and 84.7% over an earlier period, 11-12 December 2020, where there were only 890 orbiting satellites. The deployment of more satellites into their designed orbit resulted in a higher frequency of satellite visits (and revisits) over the area. These levels, however, should be contrasted with 97.9% and 99.5% for Toronto, Canada (TC) where trials are ongoing. The TPZS of 2.9 hours in QC and MC is spread across numerous times in the 24-hour period, and stands in stark contrast to the 5.97 minutes in TC where they are fewer and farther between. Despite the PDZS of 2.9 minutes not being too far from 1.47 minutes between the locations, the number of zero satellite instances in QC and MC over the course of the day are rather high to make for a seamless connectivity experience. This is expected to improve, however, with a further increase in the number of orbiting satellites in the constellation.
We must emphasize that “availability” in this context means at least one satellite being visible over the chosen Field of View (FOV). While this may be a necessary condition, it is not sufficient to guarantee connectivity to the Internet. As in any satellite telecommunication network, the connectivity follows a “bent pipe” configuration. Signals that go from the ground terminal to the satellite (i.e. the uplink) should be matched with a corresponding link from the satellite to another point on the ground (i.e. the downlink) where the facilities that connect to other terrestrial infrastructure and, eventually, the Internet, exist. This facility is called a “Gateway” (GW) and, as the name suggests, serves as the interface or interconnection point from the satellite network to the rest of the world. Currently, the Starlink GWs are concentrated in areas where the initial trials are being conducted, e.g. North America. Trials in Australia are slated or being reported, however, which imply that GW facilities in the Asia-Pacific and Oceana region may be in the offing. The GW is necessary to complete the satellite Internet network and it is imperative to have one or more located within the coverage or footprint of the satellites. More GWs within the footprint helps improve network performance through path diversity, e.g. for mitigating rain fades that can cause outage.
Neither is the presence of one or more satellites within the FOV a complete indicator of the Internet experience, e.g. the speed and/or latency of the connection. The throughput in terms of Megabits per second (Mbps) or even Gigabits per second (Gbps) depends on the amount of capacity available to the user. This, in turn, depends on several factors such as the signal level or general quality of the radio link, which may be degraded by noise and interference. Moisture or water droplets as well as buildings and other obstructions along the transmission path can attenuate the radio signal and lead to periods of deep fade. The subsequent weak reception makes the communication vulnerable to errors, which may require retransmissions that degrade the throughput. Latency, on the other hand, is the delay incurred by the data in getting from source to destination. This is affected by the distance of from the ground to the satellite (where LEO satellites have an advantage since they are closer to the Earth), the number of “hops” between satellites until the nearest GW [f]It is reported that Starlink satellites will support a feature that allows communication directly between satellites through the use of high speed optical (i.e. laser) links. and then the routing from the GW to the final destination on the Internet. Latency is also influenced by retransmissions, i.e. the need to keep repeating the same transmission because it was not properly received slows down the overall connection.
Finally, we note that the current 1,017 satellites is still quite a ways from the 12,000 reported as the final size of the first generation constellation. With SpaceX regularly launching rockets and deploying Starlink satellites with success, there seems to be steady progress towards that goal. This progress also entails improving the availability over areas in the Philippines such as Quezon City and Marawi City, eventually reaching the levels “seen” over Toronto, Canada where trials are being conducted towards full-fledged commercial service. With improving coverage over the country, the formal entry of Starlink can follow closely behind. Under current regulatory policies of the country, an entity setting up telecommunications infrastructure and a public utility requires 60% Filipino ownership and a Congressional franchise. The reduced ownership stake can be a major consideration for companies making large capital outlay investments as it is likely to impact cost recovery. Starlink can opt to offer their satellite Internet service through an already enfranchised local entity[g]Talks with Converge, a local Internet Service Provider, have been reported by the media.. In such a case, it is the local entity that resells the capacity and directly interfaces with the consumers. It thus takes more than just the number of available satellites overhead to be able to realize Starlink Internet service locally.
But – yes – go ahead and sign up for that Starlink service reservation – we know you are ready.
Whether they are ready for us in our neck of the woods, it seems that there are still a few more hoops to jump through.
How to cite this article:
Marciano, J. S. Jr., Salces, A. C., and Paler, H.B.S. (17 March 2021). Simulating Starlink “Availability” Over the Philippines. Philippine Space Agency. http://philsa.gov.ph/news/a-simulation-of-starlink-satellite-availability-over-the-philippines/
Article credits:
Dr. Joel Joseph S. Marciano, Jr.
Philippine Space Agency (PhilSA)
https://twitter.com/PhilSADG/status/1373812291963998210
Dr. Adrian C. Salces
STAMINA4Space (S4S) Program,
University of the Philippines Diliman (UPD)
Mr. Harold Bryan S. Paler
Advanced Science and Technology Institute,
Department of Science and Technology (DOST-ASTI)
Annex
Here is a step by step guide explaining how you can use Orbitron to track the Starlink satellites or any other orbiting satellites. Doing this would enable you to know exactly when a satellite will pass over your location.
-
- Download and install the Orbitron software from this URL: http://www.stoff.pl/.
- Input the name and coordinates of your location in the Location tab and click [Add to list].
- You need to frequently update the Two Line Elements (TLE) in order to have an accurate representation of the satellites’ orbits around the Earth. To do this, you need to:
- Right click on the Main Window.
- Select [Viewport] >> [Configure].
- This will download all the updated TLEs of the satellites and will update the list in the file Active.txt.
- The Active.txt file contains all the active satellites that are currently orbiting our planet.
- Click Apply.
- After updating the TLEs, we will now load the TLEs into the software. To do this, click on the [Load TLE] button on the right and select the active.txt file. This will display all the satellites on the list.
- After updating the TLEs, we will now load the TLEs into the software. To do this, click on the [Load TLE] button on the right and select the active.txt file.
- This will display all the satellites on the list. can click on all the Starlink satellites on the list or any satellite that you wish to track.
- You can also select the Simulation option and project the location of the satellites at a different date and time.
- Feel free to explore all the other features of the software and gain more insights on how satellites behave in space.
References
[1] “Application for Fixed Satellite Service by Space Exploration Holdings, LLC, SAT-MOD-20200417-00037 / SATMOD2020041700037 – Attachment Narrative App”, US Federal Communications Commission, 2020, https://fcc.report/IBFS/SAT-MOD-20200417-00037. Accessed 7 March 2021. [2] “Application for Fixed Satellite Service by Space Exploration Holdings, LLC, SAT-LOA-20170301-00027 / SATLOA2017030100027 – Attachment Legal Narrative”, US Federal Communications Commission, 2020, https://fcc.report/IBFS/SAT-LOA-20170301-00027. Accessed 7 March 2021. [3] “Deployment of 60 Starlink satellites confirmed”, SpaceX Twitter page, 6 October 2020, https://twitter.com/elonmusk/status/1313462965778157569?ref_src=twsrc%5Etfw%7Ctwcamp%5Etweetembed%7Ctwterm%5E1313462965778157569%7Ctwgr%5E&ref_url=https%3A%2F%2Fwww.tesmanian.com%2Fblogs%2Ftesmanian-blog%2Fstarlink-tibro. Accessed 7 March 2021. [4] “SpaceX Starlink receives license to offer satellite internet in Australia”, Tesmanian, Evelyn Arevalo, 12 October 2020, https://www.tesmanian.com/blogs/tesmanian-blog/starlink-tibro. Accessed 7 March 2021. [5] “Application for Fixed Satellite Service by Space Exploration Holdings, LLC, SAT-LOA-20200526-
00055 / SATLOA2020052600055 – Attachment Narrative App”, US Federal Communications Commission, 2020, https://fcc.report/IBFS/SAT-LOA-20200526-00055. Accessed 7 March 2021. [6] “SpaceX Starlink has some hiccups as expected, but users are impressed”, Ars Technica, Jon Brodkin, 13 November 2020, https://arstechnica.com/information-technology/2020/11/spacex-starlink-has-some-hiccups-as-expected-but-users-are-impressed/. Accessed 7 March 2021. [7] “SpaceX finally launches Starlink v1.0 Launch 12”, NASA Spaceflight, Danny Lentz, 5 October 2020, https://www.nasaspaceflight.com/2020/10/spacex-twelfth-launch-starlink-v1-0/. Accessed 7 March 2021. [8] “NTC reports improvement in mobile, internet services”, Manila Standard, Darwin Amojelar, 8 December 2020, https://www.manilastandard.net/index.php/business/it-telecom/341539/ntc-reports-improvement-in-mobile-internet-services.html. Accessed 7 March 2021. [9] “Orbital Slots and Space Congestion”, Space Legal Issues – 2021, Louis de Gouyon Matignon, 8 June 2019, https://www.spacelegalissues.com/orbital-slots-and-space-congestion/. Accessed 7 March 2021. [10] “The Space Legal Issues with Mega-Constellations”, Space Legal Issues – 2021, Louis de Gouyon Matignon, 3 November 2020, https://www.spacelegalissues.com/mega-constellations-a-gordian-knot/. Accessed 7 March 2021. [11] “Starlink space display ‘set to continue all week’”, BBC News, 20 April 2020, https://www.bbc.com/news/uk-england-52355706. Accessed 7 March 2021. [12] “SpaceX to make Starlink satellites ‘invisible’ after light pollution complaints from astronomers”, Sky News, 7 May 2020, https://news.sky.com/story/spacex-to-make-starlink-satellites-invisible-after-light-pollution-complaints-from-astronomers-11984439. Accessed 7 March 2021. [13] “Application for Fixed Satellite Service by SpaceX Services, Inc., SES-LIC-20190211-00151 /
SESLIC2019021100151 – Attachment App. Narrative”, US Federal Communications Commission, 2020, https://fcc.report/IBFS/SES-LIC-20190211-00151. Accessed 7 March 2021.
Footnotes
↑a | Ku-band and Ka-band are designated portions of the electromagnetic spectrum with a range of frequencies within 12-18 GHz and 26.5-40 GHz, respectively. |
---|---|
↑b | V-band is within the range of 40-75 GHz. |
↑c | Includes the phase array antenna, satellite modem, mount and Wi-Fi router |
↑d | CelesTrak maintains an updated online catalog of satellites and Two Line Elements sets according to the surveillance data on space objects from the North American Aerospace Defense Command (NORAD). Website: https://celestrak.com/. |
↑e | High-rise buildings, trees or other objects may come within the FOV and obstruct the signal path as the ground terminal antenna tracks the passing satellite. Choosing a wider FOV, e.g. 25° minimum elevation, increases that likelihood. Our simulations do not account for such possibilities. |
↑f | It is reported that Starlink satellites will support a feature that allows communication directly between satellites through the use of high speed optical (i.e. laser) links. |
↑g | Talks with Converge, a local Internet Service Provider, have been reported by the media. |